INTRODUCTION
In 2025, South Korea is entering an ultra-aged society where the elderly population comprises over 25% of the total population.1 Major health threats to the elderly include chronic conditions such as hypertension, diabetes, cardiovascular diseases, and arthritis, as well as cognitive impairments like dementia and depression.2 Among these, dementia stands out as a debilitating condition, primarily afflicting the elderly. It is a neurodegenerative disease affecting the central nervous system, impairing cognitive functions such as memory, reasoning, judgment, and emotional control, significantly impacting daily life.3
The causes of dementia are diverse, with aging and genetic factors increasing the risk of onset. Unhealthy lifestyles and chronic diseases are also strongly associated with the development of dementia. Given the absence of a complete cure, prevention and early diagnosis are important.4 Maintaining a healthy lifestyle, including regular physical activity and exercise, plays a vital role in dementia prevention.5 Exercise helps sustain healthy brain function, alleviates neurodegeneration due to aging, and enhances cognitive abilities and memory.6 Various forms of exercise have proven effective in dementia prevention, with aerobic and cognitive exercises garnering attention in academia.6
Aerobic exercise increases blood flow to the brain, supplying oxygen and nutrients, thereby reducing the risk of dementia.7 In clinical exercise therapy for individuals with ischemic conditions, maintaining an upright posture during exercise may be challenging due to reduced blood flow to the brain. To address this issue, a recumbent cycle was developed in 2017, allowing individuals to engage in aerobic exercise while minimizing the effects of gravity, particularly in rehabilitation centers for vascular diseases in large hospitals.8
The common carotid artery (CCA) bifurcates into the internal carotid artery, supplying blood to the brain, and the external carotid artery, providing blood to the scalp and face. Assessing the blood flow in the common carotid artery is important for evaluating brain health. Doppler ultrasonography is a non-invasive method to measure the thickness of the intima and media layers of the common carotid artery, peak systolic flow velocity (PSV), and pulsatility index (PI), offering valuable insights into the smooth flow of blood to the brain and the extent of vascular sclerosis.9,10
Changes in posture can influence cerebral blood flow. Therefore, understanding how changes in body position affect peak systolic flow velocity and pulsatility index is essential for determining exercise intensity in patients with vascular-related cognitive impairments, including ischemic vascular dementia. Developing an exercise protocol that considers body position is particularly relevant for recumbent cycle exercise programs, as it can help prevent the potential side effects of reduced blood flow in the common carotid artery associated with exercise therapy in ischemic conditions.
Existing research has primarily focused on analyzing data related to cardiovascular responses during stationary bicycle or treadmill exercises, emphasizing variables such as electrocardiography, respiratory gases, and blood pressure.11,12 Studies measuring changes in cerebral blood flow during exercise have typically used mathematical calculations based on altered blood pressure before and after exercise.13-15 However, there is currently a lack of research investigating changes in common carotid artery peak systolic velocity, pulsatility index, and rate of perceived exertion during recumbent cycle exercise in relation to body posture.
This study aims to investigate how trunk postures at 100°, 130°, and 160° angles during recumbent cycle exercise, which has gained popularity in rehabilitation settings for patients with ischemic conditions, affect the common carotid artery’s peak systolic velocity, pulsatility index, and perceived exertion. This study hypothesizes that changes in body posture will influence common carotid artery peak systolic velocity, pulsatility index, and perceived exertion.
METHODS
The criteria for selecting participants in this study are as follows for inclusion criteria: 1) Adults aged 19 and above who have never experienced recumbent cycle exercise, 2) have no history of being treated for hyperlipidemia or coronary artery disease, and 3) do not experience pain in the ankles, knees, and hip joints during exercise. In addition, 1) Individuals with a history of vascular diseases, 2) those experiencing pain in the ankles, knees, and hip joints during exercise, 3) individuals with arthritis, and 4) those finding discomfort in undergoing ultrasound examinations of the common carotid artery were excluded from the study.
After conducting a preliminary experiment, the calculated sample size of 12 was obtained using the G*Power program (ver. 3.1.2; Franz Faul, University of Kiel, Kiel, Germany) with the following parameters: power (0.80), alpha level (0.05), and effect size (0.5). Considering potential dropouts, the final sample size for the study was set at 15 participants. Consent was obtained from individuals who expressed voluntary willingness to participate and demonstrated an understanding of the purpose and methods of the study (Table 1). This study received approval from the Hoseo University Bioethics Committee (Approval No: 1041231-230816-HR-167).
During recumbent cycle exercise, participants assume three different trunk postures, as illustrated in Figure 1:
-
1) 100° Trunk Posture (100° TP): Sitting with a trunk angle of 100 degrees at the hip joint.
-
2) 130° Trunk Posture (130° TP): Leaning against the backrest with a trunk angle of 130 degrees at the hip joint.
-
3) 160° Trunk Posture (160° TP): Leaning against the backrest with a trunk angle of 160 degrees at the hip joint.
To determine standardized exercise training intensity, considering variations in weight and fitness levels among participants, exercise intensity was determined using the tablet computer software connected to the recumbent cycle. The maximal exercise load test started at a load of 10 watts with a speed of 60 revolutions per minute (RPM), and the protocol involved an automatic increment of 10 watts per minute. The exercise test was terminated if the participant stopped due to fatigue or failed to maintain a speed of 60 RPM for more than 10 seconds despite maximal effort. The load at the endpoint was recorded as the Maximum Workload (MWL), and exercise intensity was determined as a percentage (%) of MWL.16
After completing the exercise according to the experimental procedure, the participant rested while the ultrasound examination equipment (Ultrasound Q30, SG Healthcare Co Ltd., Korea) with an integrated Doppler mode was used to measure the peak systolic velocity and pulsatility index of the left common carotid artery (Figure 2). The participant’s perceived exertion at the end of the exercise was measured using the Borg scale. During data measurement, the examiner positioned the participant on the examination table with the head turned 45° to the right from a supine position. The left common carotid artery was located using a probe with a central frequency set at 5.5 MHz, and measurements were conducted following standardized ultrasound examination guidelines.17
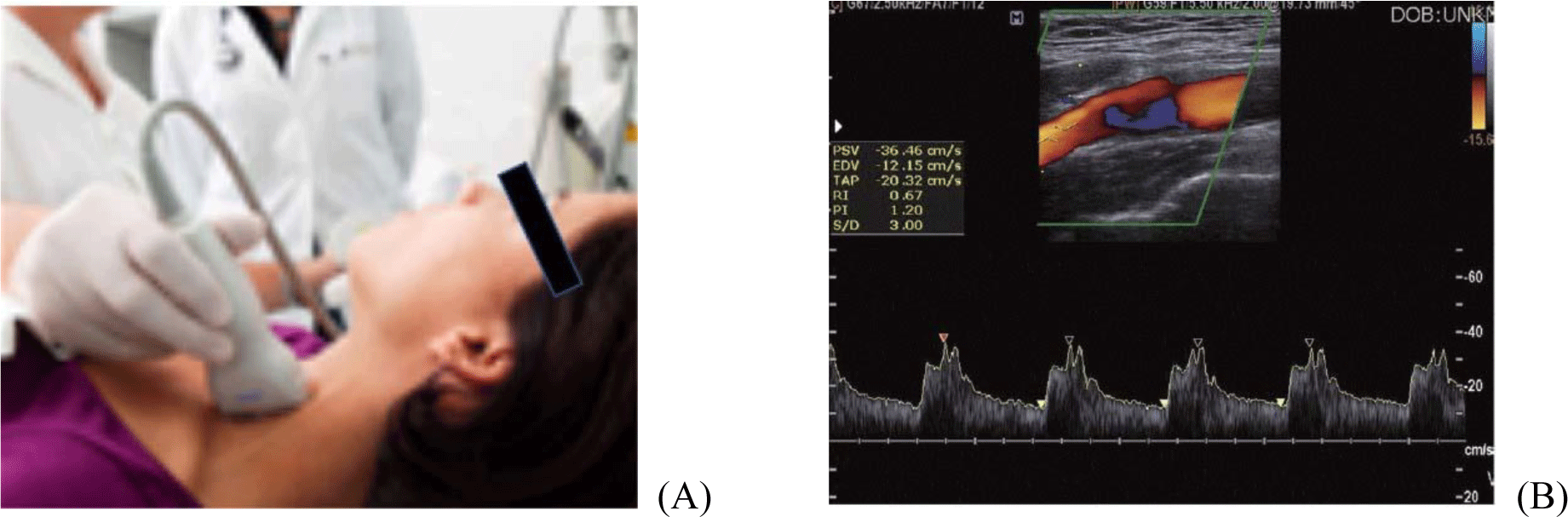
Exercise execution and data measurements were carried out by explaining the protocol to participants in advance. The following sequence of exercises and measurements was repeated as part of the experimental procedure:
Participant gender and age were obtained through a question-and-answer format, while height and weight were measured using the InBody body composition measurement device (Table 1). After collecting personal characteristic data, participants were positioned in a physiotherapy bed, and resting heart rate and blood pressure were measured following an explanation of the recumbent cycle exercise method.
To standardize exercise intensity considering variations in weight and fitness among participants, a recumbent incremental maximal exercise test was conducted to determine exercise intensity tailored to each participant’s muscle strength. The test, administered through an automated program integrated with the recumbent cycle equipment and tablet computer, lasted for 10 minutes. After the test, participants were allowed to sit in a chair and rest for 10 minutes.
Participants performed a recumbent cycle exercise in one randomly assigned posture among the three. The specific structure of the individualized exercise program involved pedaling on the recumbent cycle at a fixed speed of 60 RPM with a load equivalent to 40% of the Maximum Workload (MWL) for 15 minutes. Perceived exertion was measured using the Borg scale at the end of the exercise.
After completing the exercise, participants rested in a supine position on a physiotherapy bed, and the researcher used ultrasound equipment to measure the left common carotid artery blood flow velocity and pulse index.18,19
Participants rested in the physiotherapy bed until their heart rate and blood pressure returned to baseline. The entire procedure, including recumbent cycle exercise and data measurements, was then repeated for the remaining two trunk postures in a randomized order. This systematic approach ensured a comprehensive evaluation of the impact of different trunk postures on physiological responses during recumbent cycle exercise.
The data obtained from the experiment were analyzed using SPSS version 25.0 to examine differences among trunk posture conditions. To compare the mean values of the data measured in the three different trunk postures, a one-way repeated measures analysis of variance (ANOVA) was applied. When a significant difference was observed in the results based on the posture changes (p&0.05), Bonferroni post-hoc tests were conducted to assess the significance (α= 0.017). This statistical approach allowed for a comprehensive exploration of the impact of various trunk postures on the measured variables and facilitated the identification of significant differences between the conditions.
RESULTS
Statistically significant differences were observed in the mean values of Peak Systolic Velocity (PSV) and Borg scale scores among the three trunk postures: sitting with a trunk angle of 100 degrees at the hip joint (100° TP), leaning against the backrest with a trunk angle of 130 degrees at the hip joint (130° TP), and leaning against the backrest with a trunk angle of 160 degrees at the hip joint (160° TP) during recumbent cycle exercise (p&0.05). However, there was no statistically significant difference in the mean value of the Pulsatility Index (PI) (p=0.533) (Table 2). This suggests that the choice of trunk posture during recumbent cycle exercise has a significant impact on both blood flow velocity and perceived exertion, while it does not significantly affect the Pulsatility Index.
In the Bonferroni post-hoc analysis, Peak Systolic Velocity (PSV) exhibited statistically significant differences between 100° TP and 160° TP (p=0.001), as well as between 130° TP and 160° TP (p=0.001). However, there was no statistically significant difference between 100° TP and 130° TP (p= 0.729) (Figure 3). For the Borg scale, significant differences were found between 100° TP and 160° TP (p=0.001) and between 130° TP and 160° TP (p=0.001). However, there was no statistically significant difference between 100° TP and 130° TP (p=0.626) (Figure 3). These results suggest that the choice of trunk posture significantly influences both PSV and perceived exertion during recumbent cycle exercise, with specific differences observed between certain posture pairs.
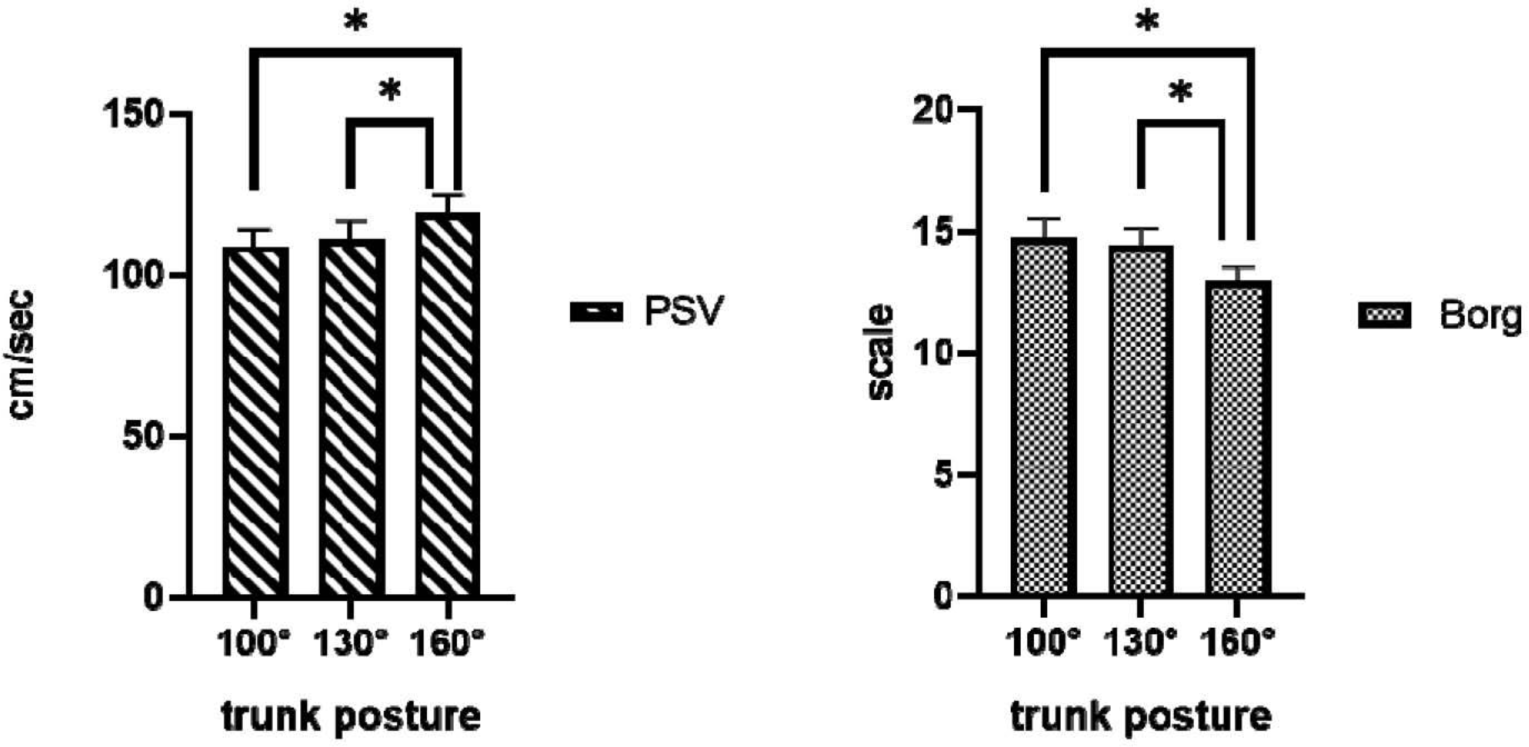
DISCUSSION
The hypothesis of this study posited that during recumbent cycle exercise, ‘changes in trunk posture would lead to alterations in peak systolic velocity (PSV) of the brachial artery, pulse index (PI), and perceived exertion.’ The results showed that as the trunk angle changed from 100° to 130° and 160°, the PSV increased by 2.20% and 9.63%, respectively, while perceived exertion decreased by 2.24% and 12.22%. These differences were statistically significant, partially supporting the research hypothesis. However, there was no statistically significant change in PI.
The increase in PSV with a more reclined trunk posture suggests enhanced venous return due to decreased gravitational effects on limbs and head, leading to increased preload. Such hemodynamic advantages align with the clinical application of recumbent cycle exercise in cardiac rehabilitation. Additionally, for elderly individuals with arterial stiffness, recumbent cycling may serve as a method to delay disease progression and prevent vascular dementia. However, the study noted that the difference in speed between 100° TP and 130° TP was not statistically significant, indicating the need for further investigation into the specific angle required for optimal hemodynamic benefits.20
Although PI exhibited a slight increase with changes in trunk posture, the lack of statistical significance suggests that the participants, being young and healthy, could adequately handle the increased blood flow without a significant change in this parameter. However, caution is advised in extending these findings to older individuals with altered vascular elasticity and increased intimal thickness.21 The study found that perceived exertion decreased with a more reclined trunk posture, which aligns with previous research suggesting reduced muscle fatigue and delayed glycogen depletion in a more recumbent position. The results indicate the potential for recumbent cycle exercise, especially at a 130° trunk posture, to be recommended as a preventive method for dementia in the elderly.22
This study investigated the changes in brachial artery peak systolic velocity (PSV), pulse index (PI), and perceived exertion associated with three different trunk postures during recumbent cycle exercise. The results revealed that as the trunk posture became more reclined, PSV increased, with 160° TP showing statistically significant differences compared to the other two angles. Conversely, perceived exertion decreased with a more reclined trunk posture, and 160° TP demonstrated statistically significant differences compared to the other two angles. Based on these findings, recumbent cycle exercise for increased cerebral blood flow is recommended in the order of 160° TP followed by 130° TP. The outcomes of this study provide valuable scientific insights into the interaction between trunk posture and hemodynamic variables during recumbent cycle exercise. By identifying specific trunk postures that can alter cerebral blood flow, this research contributes to the development of recumbent cycle exercise programs aimed at preventing vascular dementia in the elderly and promoting brain health in individuals with ischemic conditions.21,22
The study acknowledges several limitations. Firstly, it only considered three trunk postures, and the specific threshold angle for significant changes in PSV and perceived exertion was not identified. Secondly, the lack of statistical significance in PI changes might be attributed to issues such as examiner reliability and participant learning effects. Thirdly, the study’s sample consisted of individuals in their 20s, limiting the generalizability of the findings to older populations and the small sample size. To address these limitations, future research should include a broader range of trunk postures, employ more comprehensive vascular measures, and focus on elderly participants and the large sample size with diverse health conditions to establish more robust clinical guidelines for recumbent cycle exercise in dementia prevention.
CONCLUSIONS
The study results indicate that trunk posture plays an important role in recumbent cycle exercise, particularly when aiming to modify cerebral blood flow. These findings can provide a basis for developing exercise interventions that prioritize brain health for the aging population and people with ischemic disorders. Ultimately, this study’s information can inform the design of effective recumbent cycle exercise programs that aim to prevent vascular dementia and improve overall brain health.